The Qubit zoo
There’s many different ways to build a qubit—but choosing the right approach depends on what we want to do with it.
The fundamental building block of classical computers, from smartphones to supercomputers, is the bit. Bits are built out of semiconductor-based devices called transistors. They are a feat of modern engineering: billions of transistors can be fabricated on a single chip and are operated with tremendous reliability.
In contrast to classical computers, we are currently still in the early stages of the development of quantum computing, and there is no “best way” to build a qubit. But there are many successful approaches, each with their own advantages.
Superconducting Qubits
Superconducting qubits require three ingredients: (1) cold electronic circuits, (2) superconducting material, and (3) so-called “Josephson junctions”.
Extremely cold electronic circuits behave quantum mechanically. By constructing these circuits out of a superconducting material – a material with no electrical resistance – the tiny currents in these circuits last long enough that they can be controlled. Small gaps in the circuit called Josephson junctions are required to isolate the two distinct “0” and “1” states used to form a qubit.
There are actually many types of superconducting qubit, the most successful of which has been the “transmon” which was used in Google’s famous quantum supremacy experiment in 2019. Devices with 50-100 qubits are currently being prototyped, making them the most commercially advanced qubit to date. Yet, they have a relatively large footprint, and it is not clear if their coherence time can still be significantly improved.
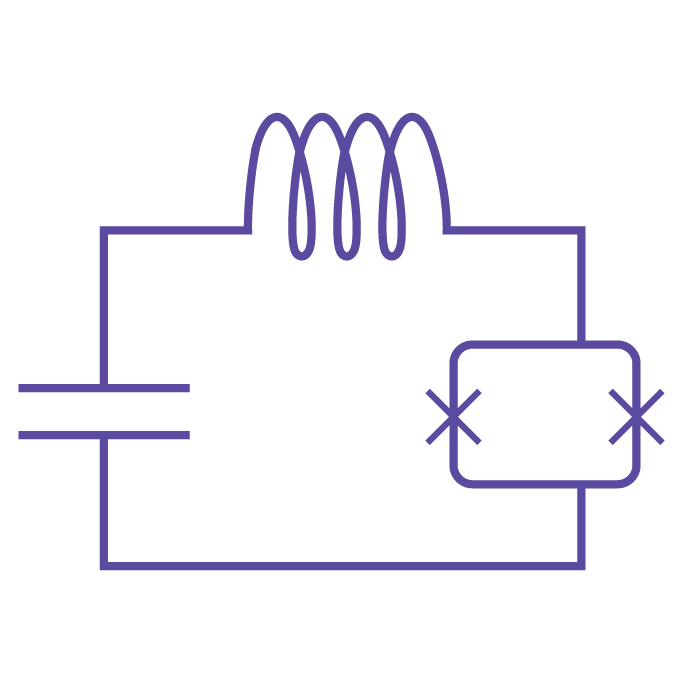
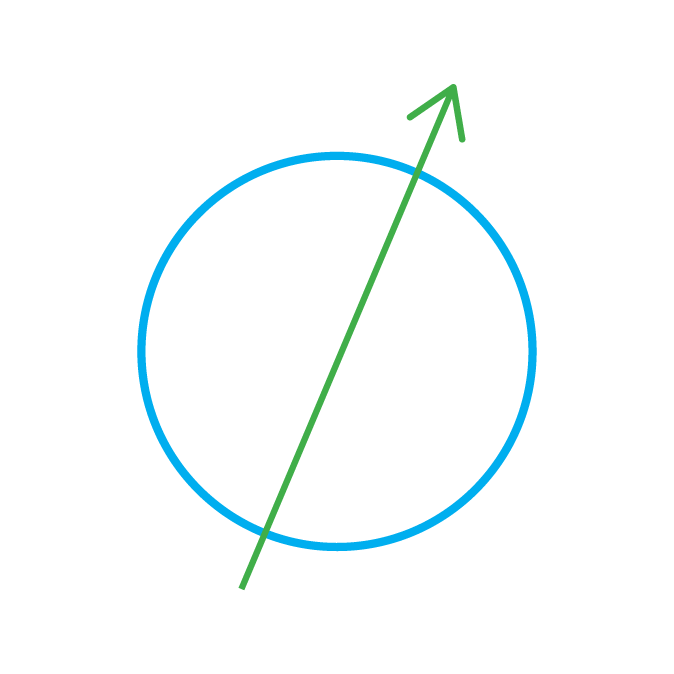
Spin Qubits
The spin of an electron is the quantum property that makes it behave like a tiny magnet. In certain materials, like iron, many of these spins add together to make things like fridge magnets and compasses.
An individual spin is, by several measures, the ideal qubit. When a spin is placed in a magnetic field, it exists in two states: aligned with the field or against the field. The challenge is to trap the individual electrons which hold the spin. One method involves confining electrons in very small semiconductor boxes called quantum dots. This can be done on silicon chips that closely resemble the chips used in classical computers. It is hoped that the advanced fabrication techniques used by the semiconductor industry will be able to manufacture the millions of qubits necessary to build a world-changing quantum computer. It is still a challenge though to connect many spin qubits. Another technique to capture individual spins requires defects such as so-called NV centers in diamond. Despite the name, these defects are quite useful for trapping single electrons in an otherwise perfect crystal and make for high-quality qubits.
A useful property of the NV center is that it allows a spin qubit to be converted into a photonic qubit, allowing to transfer the qubit over an optical fiber. It remains challenging to scale to very many qubits, though.
Ion Traps
Ions are electrically charged atoms, meaning they are particles found on the periodic table of elements that have lost or gained electrons. All ions of the same element and charge are indistinguishable – a calcium ion in Amsterdam is the same as one on Mars. Since ions are charged, they may be “trapped” using a combination of electric fields which confine a group of ions in a small vacuum chamber. The atoms may then be laser cooled to extremely low temperatures, such that they form a chain of ions. Ions are tiny, and quantum mechanical by nature. To make qubits, ion trappers pick a particular ion, such as Ytterbium-171, that has two high-quality quantum states. The qubit formed by these two states can be controlled with precise laser pulses, and qubits can interact with one another through vibrations in the ion chain. Trapped ions have achieved the best-quality logical gates of any qubit type, but again it remains challenging to scale to many qubits.
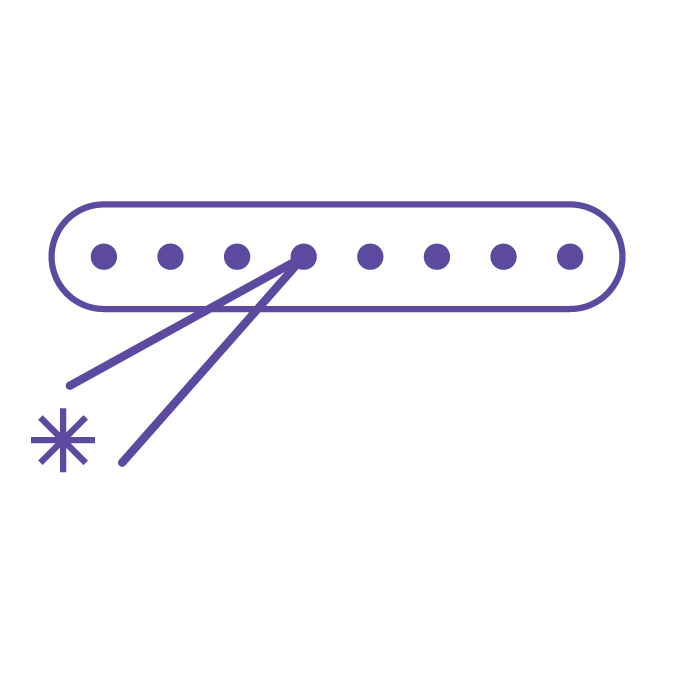
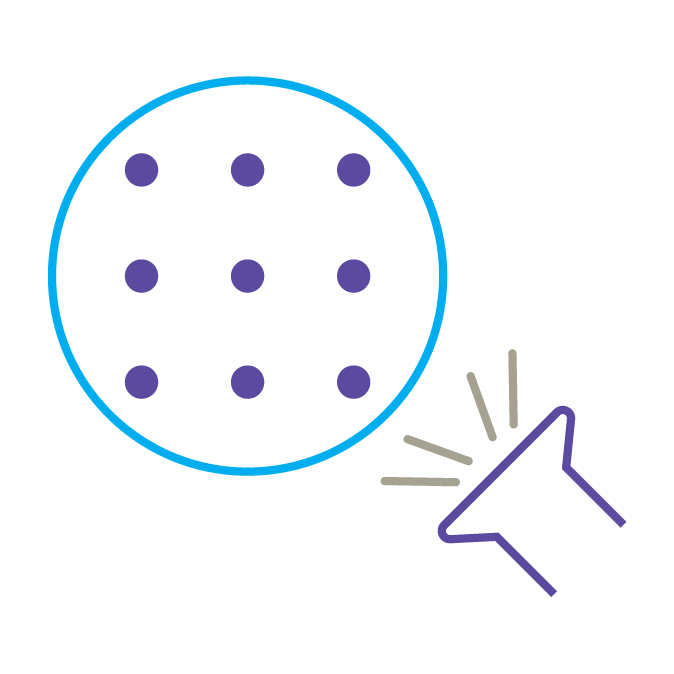
Neutral Atoms
Neutral atoms share some notable similarities with trapped ions, but show also many key differences. Similar to a trapped ion, a neutral atom can form a qubit by selecting two high-quality quantum states of the atom. Quantum computation can be performed by manipulating the neutral atoms with lasers, just as with trapped ions. However, unlike ions, neutral atoms are not charged, meaning they can’t be electrically trapped in the same way as ions. Instead, neutral atoms are trapped using precisely focussed light beams called optical tweezers which can move individual atoms around in a vacuum. Hundreds of neutral atoms can be arranged intricately in three dimensions, making neutral atoms a very versatile platform. The main challenge for this type of qubit is the comparatively low accuracy of gate operations.
Photons
One very important consequence of quantum mechanics is that light comes in discrete packages of energy called photons. Photons, unlike electrical circuits, atoms or spins, are always moving at an incredibly high speed – nearly 300 million meters per second in vacuum. This inspires the name “flying qubits”, often associated with photons, and it makes them ideal candidates for moving quantum information around in a network of quantum devices. There are many ways to make a qubit using photons. One common approach is to use the photon’s polarization, or orientation, which may be in the “horizontal” or “vertical” state. Just like polarizing sunglasses are used to filter out sunlight, photon detectors can be designed to measure only certain polarized quantum states. Other special crystals called wave plates and beam splitters, can be used to “rotate” photon polarization and conditionally manipulate their trajectory. Currently, loss of photons, and thus of qubit states, is most limiting for this technology, making scaling-up an ongoing challenge.
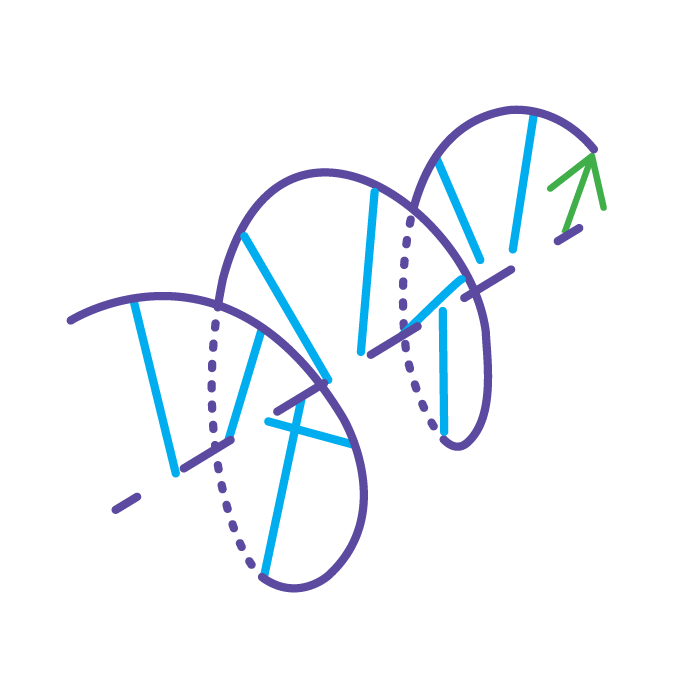
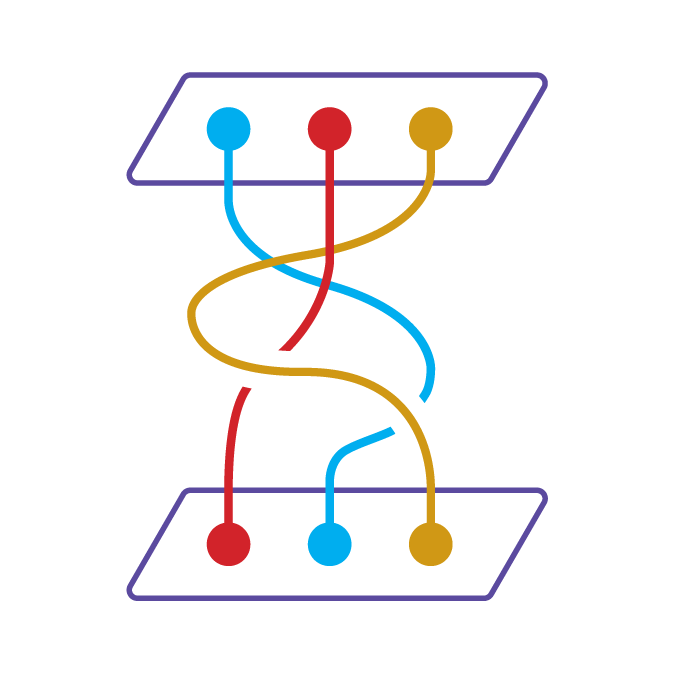
Topological Qubits
Consider an elastic band. Regardless of how you stretch, twist, tie, or scrunch the elastic band, it will always contain a single loop (as long as we don’t break it, which isn’t allowed). We can call this loop a topological property of the elastic, since it is robust to many types of deformation.
Topological qubits strive to take advantage of similar robustness. Imagine you have two special particles on a flat surface. You might reasonably expect that dragging one particle in a loop around the other won’t change anything. However, at the quantum scale, braiding one particle around the other results in a measurably different state, and this type of operation can constitute the building blocks of a topological quantum computer. The exact details of the path, – the zigs and zags the particle took on its journey, – don’t matter, similar to how scrunching the elastic band doesn’t change the fact that it’s a loop.
This property makes topological qubits constructed out of these sorts of particles very resistant to errors. In practice, topological qubits are challenging to engineer, and many aspects are still in the stage of fundamental science. However, the allure is tremendous: a quantum processor constructed out of topological qubits would likely require fewer qubits and be more efficient than quantum computers using other qubit technologies.
There are many ways of approaching the building of qubits. The next challenge is combining and preserving them.