Distinguished Dynamics of micro and nanosystems Colloquium
The distinguished dynamics of micro and nanosystems talks is a virtual platform organized by the DMN group at TU Delft aiming at inviting renowned scientists working in the cross fields of mechanics, dynamics, nanotechnology, and physics to communicate their recent findings, exchange experience, and keep the momentum of international interactions during the pandemic.
Next Talk: 31 May 2022
"Phoxonic metamaterials and microsystems" by Xavier Rottenberg, IMEC Fellow
Biography
Dr. Xavier Rottenberg obtained his MSc degree in Physics Engineering and the DEA in Theoretical Physics from the Université Libre de Bruxelles in 1998 and 1999, respectively. He obtained in 2008 his PhD degree in Electrical Engineering from the KU Leuven. He worked one year at the Royal Meteorological Institute of Belgium in the field of remote sensing from Space. He has been at IMEC Leuven since 2000, where he contributes to research in the field of RF, RF-MEMS, photonics and microsystems modelling/integration. As imec fellow, he currently leads the Wave-based Sensing and Actuation Developments, working among other topics on integrated photonics, flat optics, acoustics, photo-acoustics and M/NEMS. He has (co-)authored over 150 peer reviewed publications, has been granted various patents, was a lecturer or invited speaker at DTIP (‘05), EuroSimE (‘08), ESREF (‘08), ESoA (‘10/‘12/‘14), RF-MEMS summer school (‘13), FETCH 2016, NERF Neurotechnology Symposium (‘16), OpenMinds (‘17), Nanoworkshop at Beijing University (‘18) and co-founded Pulsify Medical in 2019, a young company developing ultrasound imaging patches.
About Imec
Imec is an international research & development organization. It enables nano- and digital technology innovation with a significant impact on the quality of life. With partners from companies, governments and academia. And supported by the three pillars of R&D: (1) a unique infrastructure that includes a 2.5-billion-euro 300mm semiconductor pilot line, (2) more than 5,000 expert scientists from over 95 countries, and (3) an ecosystem of more than 600 world-leading industry partners and a global academic network. Founded in 1984, imec quickly made its name as the leading research hub for advanced CMOS scaling.
Past Talks:
Towards single-molecule analysis with nanomechanical resonators
Silvan Schmid
TU Wien
1 February 2022

About Silvan Schmid
Silvan Schmid has a strong background in nanomechanical sensing. He completed his mechanical engineering education from ETH Zurich in 2003. He performed his doctorate in the Micro and Nanosystems Group at ETH Zurich and received his doctoral degree in 2009. He got awarded with the ETH medal 2009 for an outstanding doctoral thesis. After his doctorate, Silvan Schmid started a postdoc position at the Technical University of Denmark (DTU). In 2012 he received the Villum Foundation's Young Investigator Programme grant, which supports especially talented young Danish researchers in science and technology, which allow him to independently conduct his own research as an Assistant Professor and later as Associate Professor at DTU. In 2016 Silvan Schmid got appointed Full Professor at TU Wien, where he since heads the Research Unit of Micro and Nanosensors at the Institute of Sensor and Actuator Systems. Also, in 2016, he received an ERC Starting grant to work on nanoplasmomechanical systems, and in 2019 an ERC Proof of Concept grant to develop a nanoelectromechanical infrared detector. Silvan is co-founder and scientific advisor of the TU Wien spin-off Invisible-Light Labs GmbH that was founded in 2019.
Abstract
The identification and quantification of samples are a fundamental task in most applications in medicine, science and engineering. Mass spectrometry and absorption spectroscopy are two indispensable instrumental methods available in analytical chemistry today. While these are two highly capable methods, their limit of detection is in the femtogram and nanogram range, respectively. There is a big demand for the identification of samples with ever-increasing sensitivity in emergent applications such as single-cell protein profiling or environmental trace analysis. Nanomechanical resonators have already demonstrated single-molecule mass and photothermal absorption sensitivity. In our group we work towards the realisation of single-molecule analytical chemistry with nanomechanical resonators. In this effect, we have developed a novel method based on surface acoustic waves that enables the transduction of individual nanopillars resonators for the application in single-molecule mass spectrometry. And we are exploring single-molecule photothermal absorption spectroscopy based on nanomechanical silicon nitride resonators.
Mechanically tunable strain fields in suspended graphene resonators
Christoph Stampfer
RWTH Aachen University
December 14, 2021
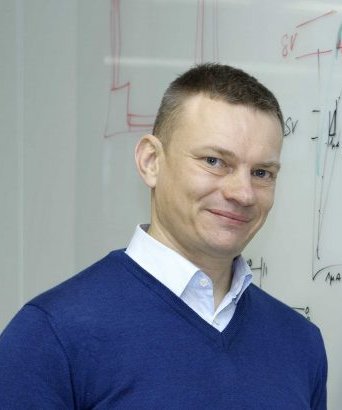
About Christoph Stampfer
Christoph Stampfer is Professor of Experimental Solid State Physics at the RWTH Aachen University and researcher at the Forschungszentrum Jülich. His primary interests include graphene and 2D materials research, quantum transport, and micro electromechanical systems. He holds a Dipl.-Ing. Degree in Technical Physics from the TU Vienna (Austria) and a Ph.D. in Mechanical Engineering from the ETH Zurich (Switzerland). He was a staff member at the Institute for Micro and Nano Systems of the ETH Zurich from 2003 to 2007 and staff member of the Solid State Laboratory (Ensslin-Group at ETH Zurich) from 2007 to 2009. From 2009 till 2013 he was JARA-FIT Junior Professor at the RWTH Aachen and the Forschungszentrum Jülich. He has been awarded with an ERC Starting Grant to work on "Graphene Quantum Electromechanical Systems" in 2011, was a member of the Young Scientist community of the World Economic Forum and received in 2018 an ERC Consolidator Grant to work on “2D Materials for Quantum Technologies”.
Abstract
Controlling strain fields in graphene not only allows tailoring mechanical properties, such as resonance frequencies or quality factors but also offers a unique way of applying valley-sensitive gauge fields acting on Dirac fermions. This leads to exceptional electromechanical properties giving rise for example to pseudomagnetic fields, valley filters or pseudo gravitomagnetic froces. Although such gauge fields have received much attention thanks to the possibility of mimicking real magnetic fields, controlling strain fields in graphene has remained a major challenge. Here we show low-temperature compatible micro electromechanical systems with integrated graphene for engineering truly mechanically tunable strain fields. Spatially resolved confocal Raman spectroscopy is used for mapping the strain fields in graphene as function of clamping geometries and tunable displacement. By controlling the clamping conditions different strain fields, including strain gradients with values around 1.4% per micrometer are obtained. Importantly, the presented approach allows also for multiple axis straining and is not exclusively limited to graphene but is also applicable to the raising number of other two-dimensional materials providing a work horse for evaluating sensor and transducer capabilities.
Self-focusing nanomechanical sensors for high-throughput detection of single viruses and nanoparticles
Selim Hanay
Department of Mechanical Engineering, and
UNAM, Institute of Materials Science and Nanotechnology, Bilkent University, Ankara, Turkey
June 29, 2021
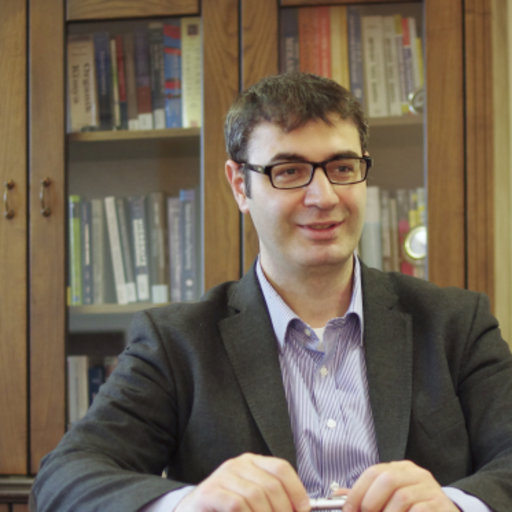
About Selim Hanay
Selim Hanay received his Ph.D. degree in Physics from Caltech in 2011 where he has developed nanomechanical sensors capable of detecting and weighing single protein molecules in real time. He continued his research at Caltech in post-doctoral capacity for several years. In 2013, he joined Bilkent University as an Assistant Professor. His current research focuses on multimode sensing with nanomechanical and microwave sensors, and the development of buckling-based actuators at the nanoscale. He is the recipient of Bilkent University Distinguished Teaching Award, as well as several national young investigator awards given by different institutions including the Turkish Academy of Science. He has been awarded with an FP7 Marie-Curie Career Integration Grant and ERC Starting Grant.
Abstract
Nano-Electromechanical Systems (NEMS) have emerged as a promising technology for the mass sensing and spectrometry of nanoparticles and viruses at the single-particle level. However, a typical NEMS device has a small cross-section area, therefore it can capture only a tiny fraction of the incoming analyte particles. As a result, the limit-of-detection and throughput of NEMS mass sensors have not yet reached the levels required to analyze realistic biologic samples. Here, we overcome this problem by integrating a NEMS sensor with an on-chip polymeric ionic lens which automatically focuses the incoming analyte ions onto NEMS. The polymeric lens charges up by accumulating part of the incoming analyte ions delivered through electrospray ionization (ESI). As charge is accumulated and maintained on the polymeric lens, a significant portion of incoming analytes are focused onto an open window on the polymer layer which is aligned with an active NEMS sensor. Owing to the increased ionic flux at the NEMS location, not only the capture efficiency is increased drastically, but also the system could be operated entirely under atmospheric conditions by eliminating the need of focusing by ion optics and the vacuum conditions needed for ion optics.
We show that different nanoparticles and viruses can be characterized rapidly, and under ambient conditions [1]. We applied this technique to obtain the mass spectrum of SARS-CoV-2 in atmospheric conditions. Moreover, we have characterized another mammalian virus, BoHV-1, as a positive control experiment. The capture efficiencies obtained here are orders-of-magnitude higher than the earlier state-of-the-art NEMS systems. The results demonstrate that on-chip integration, rather than the use of bulky and expensive hardware, can be used to solve the capture efficiency problem of nanoscale sensors. With the increase in analytical throughput, the simplicity of the overall setup, and the capability to operate under ambient conditions, the technique presented here brings NEMS mass spectrometry one step closer to operation in the field and with realistic biological samples.
[1] R. T. Erdogan, et al., “Single-Virion SARS-CoV-2 Mass Spectrometry in Air by On-Chip Focusing NEMS”, arXiv:2012.10675 (2020).
Nanotube mechanical resonators – tiny electron forces and large electron backaction
Adrian Bachtold
ICFO, The Barcelona Institute of Science and Technology, Spain
May 4, 2021
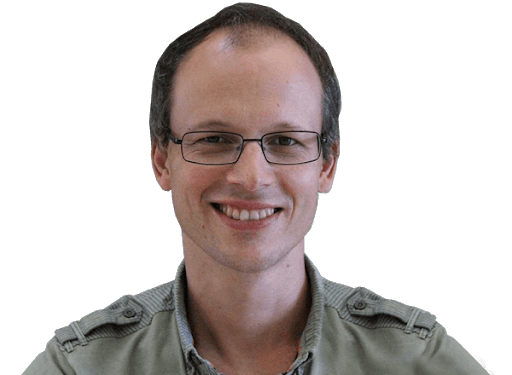
About Adrian Bachtold
Adrian Bachtold is the principal investigator of the Quantum NanoElectronics & NanoMechanics group at ICFO in Barcelona. His activities focus on the mesoscopic investigation of both quantum electron transport and mechanical resonators. The work is highly interdisciplinary with possible implications in condensed matter, quantum science, optomechanics, and low-temperature physics. He is APS fellow and is recipient of the CNRS medal and the IBM award of the Swiss Physical Society. Out of the 20 PhD students and postdoc fellows that worked in his group, 12 of them have a permanent position in academic research.
Abstract
Mechanical resonators based on carbon nanotubes feature a series of truly exceptional properties. In particular, the mechanical vibrations are highly sensitive to the tiny forces associated with the electron states in the nanotube and vice versa, leading to large backaction effects. In this talk, I will discuss our efforts to cool the amplitude of the thermal vibrations to a few quanta. Cooling is achieved using a simple yet powerful method, which consists in applying a constant (DC) current of electrons through the suspended nanotube in a dilution fridge. I will also present results where we strongly couple mechanical vibrations to the two electron states involved in single-electron tunnelling (SET). It renormalizes the resonance frequency by a large amount, up to 25% of its value. This results in a highly nonlinear potential for mechanical vibrations despite the relatively low quanta population (about 80 quanta). I will finish the presentation by explaining our effort towards the realization of a mechanical qubit.
Frontiers of nanomechanical mapping: From single ions to single cell nanomechanics
Ricardo Garcia
Instituto de Ciencia de Materiales de Madrid, CSIC, Madrid (Spain)
September 29, 2022

About Ricardo Garcia
Garcia combines theory and experiments to develop advanced force microscopes for the characterization of materials in its broader sense (inorganic, biomolecules, tissues, devices). A key feature of RG’s approach is that nanoscale control and device performance should be compatible with operation in technological environments (air or liquid). He has made major contributions to the development of the most popular AFM method (tapping mode AFM). RG has pioneered the fields of multifrequency AFM and nanomechanical force spectroscopy. He is the inventor of bimodal AFM, which is the most sophisticated nanoscale characterization tool. He has founded the Multifrequency AFM conferences. He has also contributed to the development and applications of scanning probe lithography. Currently, RG pioneers the development of 3D-AFM to characterize solid-liquid interfaces. Some of his inventions are commercialized. RG has received several awards and prizes. The most recent are the Beller lectureship by the American Physical Society (2019) and the Nanotechnology Recognition Award by the American Vacuum Society (2016). In 2013 he was awarded an Advanced Research grant by the European Research Council. He is a Fellow of the American Physical Society. He was awarded the Technology prize from the Regional Government of Madrid (Spain) in 2009.
Abstract
This contribution introduces some of the main challenges faced by force microscopy to image at subnanometer-scale spatial resolution solid-liquid interfaces. The presentation is divided in three sections. The first section is devoted to introduce the capabilities of 3D-AFM to image with atomic-scale resolution the 3D interfacial structure of surfaces immersed in aqueous solutions. The second section shows the applications of high-speed bimodal AFM to map the nanomechanical properties of several biological processes. The third section discusses some fundamental issues involving the imaging and nanomechanical characterization of live cells with the AFM.