Research
The research topics of the IEPG group can be roughly grouped into four areas:
Future-Proof Power Grids
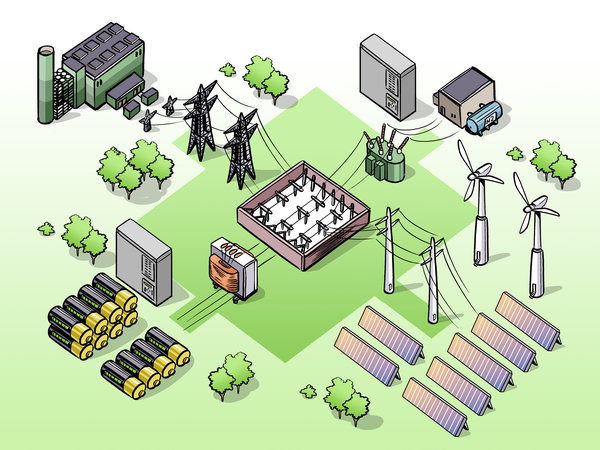
Electrical power systems in the future will significantly differ from the power systems of the twentieth century. Many coal and nuclear power plants are supposed to be replaced by variable renewable resources (VRES), mostly wind and solar energy. Due to the small capacity of VRES that can be used to supply electricity locally, the shortage of higher power should be transmitted over longer distances. Local constrain (public resistance) reduces the necessary grid expansion investments. Existing lines are operated close to their thermal and stability limits. On the other hand, because of local massive VRES the system will be operated differently in which power flows, and especially fault currents, will be bi-directional. In addition, low system inertia, uncertainty in supply because of VRES and because of "intelligent" demand of EVs and heat pumps, raises questions of how the system will be protected, monitored and operated.
Transmission networks transport large amount of renewable generation such as offshore wind. Stability requires good interconnection, which in return increases the reliability of the supply. Currently and in the future, there will be investment in HVDC as transmission of electricity on long distances is cheaper to be realized by HVDC connection. Advanced control of HVDC offers more flexibility with respect to frequency and voltage control as well as power flows.
The use of DC on medium and high voltage level raises also many challenges regarding protection and power quality issues hence the need of wide area monitoring, protection and control (WAMPAC). By decreasing the system inertia, an important subject is also how to keep the system integrated and immune to different disturbances that may cause overload and system weakness. Blackout prevention will be realized by applying synchrophasor technology for the purpose of (hybrid) state estimation, adaptive protection and load shedding, and controlled islanding. In this way, timely corrective control will be realized. As in the future power grids more VRES and smart appliances will be used, the coordination of power consumption and generation will require development of new digital platforms with complex interactions between the physical and cyber systems. We work on the grid of the future including algorithms for blackout prevention, communication platforms to interface these algorithms as well as different protection and stability studies.
Flexible Distribution Systems
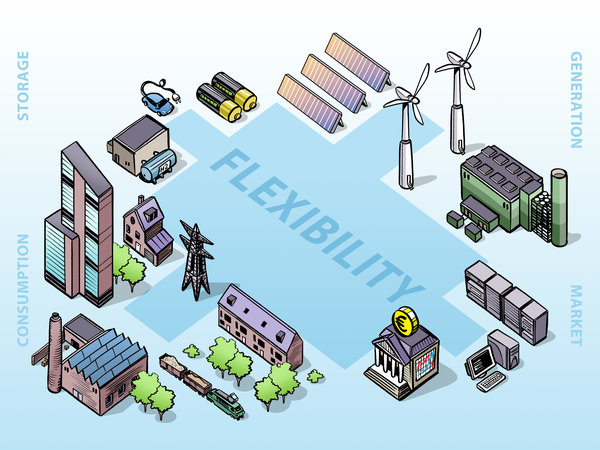
Previously, distribution system infrastructure aimed to connect the upper level (high-voltage) system to customers, while self-generation was limited to large generation units at the high-voltage system. Recently, innovations in the generation and demand side have dramatically changed the idea of distribution systems operation, giving them a more active and flexible role in the whole operation of our power system.
We understand a flexible distribution system as composed of many distributed energy resources (DERs), such as electric vehicles, electric heat pumps, PV systems, and players that actively cooperate to ensure a cost-effective and reliable operation. To ensure this, all involved players (distribution system operators, large and small customers) must coordinate their operation by adequately managing the uncertainties typical of renewable-based DERs; shifting towards a more flexible operation. These new operational conditions require introducing new control, operation, and planning models that enable the connection of new DERs without compromising the reliable operation of the distribution network. To achieve this, data is fundamental, not only from sources already available in the past (e.g., network measurement units) but also new sources coming from customers, for instance, through installing smart meters and using IoT technologies.
We have substantial expertise in using customer data to develop AI and machine learning models that can help understand, model and quantify their energy flexibility potential and enable self-organized behaviors, aiming to support the distribution network operation. Similarly, we exploit advanced digital tools such as digital twins and visualization platforms seeking to develop intelligent grid-edge technologies that ensure a distributed and optimal operation.
Integrated Energy Systems
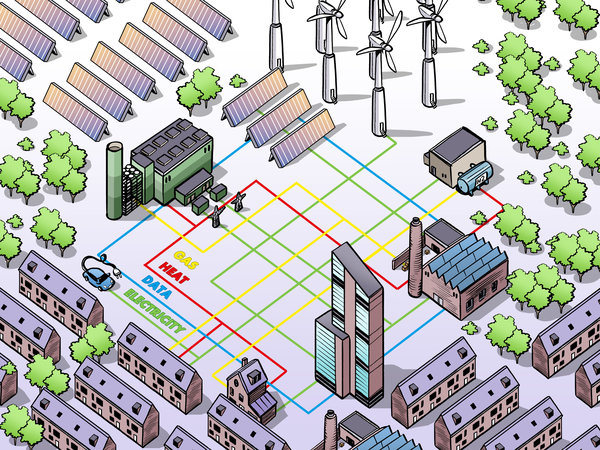
The power system interacts with a number of other systems and sectors, which have their own dynamic features. On the one hand, there are parallel energy systems for different energy carriers such as gas, heat, and fuels, and on the other hand there are environments and external processes that have an important impact on the power system, such as built environments, industrial settings, overall market and economic developments, and even societal and governmental processes. In addition, ICT plays an increasingly important role, as an enabler for future decentralized energy systems, as a consumer of electricity, and also as an important part of other systems. Understanding, describing, analyzing and optimizing such hybrid and heterogeneous systems and environments in their interdependencies is a challenge (see "Modeling & Simulation"), and so is their design and operations.
We need holistic, trans-disciplinary methods and techniques to plan and run such integrated energy systems in various and diverse environments. We work on how to connect such systems and environments and how we can identify, access and exploit the interdependencies, synergies and antagonisms that exist between them. This involves analysis and design by modeling and simulation, potentially with hardware in the loop, as well as the design of appropriate ICT approaches, coordination and managing mechanisms such as markets for supply/demand matching and ancillary services, and scheduling and planning systems for local energy management and long-term infrastructure investments. The application environments range from Smart Cities via integrated and cooperative industry parks up to cross-country mechanisms.
Reliability and Resilience

Electrical power systems are undergoing a major structural change in generation, transmission, and distribution. This change involves massive integration of power electronic converter based components to interface variable renewable energy based power plants, consumers, and different regions. Such changes are translated into unprecedented operating conditions and steady-state and dynamic performance. The roots of these changes shall be properly understood to design robust control measures to enable the transition towards 100% power supply from predominantly variable renewable energy. A future system with properly designed controls will be able to securely cope with diverse operation conditions and disturbances.
We develop probabilistic models to understand the growing stochastic nature of generation and demand. These models are key for reliability assessment of electrical power systems (involving generation, transmission, and distribution). This also involves developing models of power electronic converter based components, failures, faults, forecasts of demand (including prosumers) and renewable power generation, and sampling of operational scenarios. The probabilistic models constitute the base for the development of computationally efficient algorithms for risk-based systemic (e.g. interconnected power system) reliability assessment, taking into account the interdependencies of different reliability management tasks across different time horizons.
We also conduct research on the study of dynamic phenomena of systems with high penetration of renewable energy and responsive prosumers. This involves a number of activities, ranging from the development of models for power electronic converted based components and dynamic equivalent models for RMS and EMT simulations, up to the design of optimal and adaptive controls to mitigate instability threats. We have vast experience in conducting stability studies based on real-time digital simulation and hardware-in-the loop (RTDS-HIL). The development of the North Sea Transnational Offshore Infrastructure, the interplay transmission-distribution-microgrids, and the provision of ancillary services in the context of multi-energy conversion are among key study cases.